Throughout the vast expanses of the Universe, astronomical objects such as planetary systems, star clusters, nebulae, and galaxies take a variety of forms, painting the night sky. All of these objects, including those whose effects are observable through special equipment, are made of matter. The same fundamental particles that construct Earth and all of its flora and fauna are responsible for the massive physical entities observed and described by the likes of physicists and astronomers.
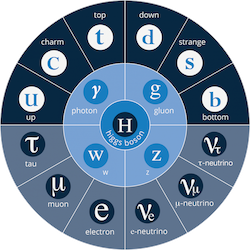
The Standard Model of particle physics is a mathematically-rigorous theory of quantum physics that describes the fundamental building blocks of all matter. For easier interpretation, it is often portrayed as a table in grid format or with concentric circles (above). It has two main groupings: fermions (matter particles — outer ring) and bosons (force particles — inner ring and central Higgs boson). Despite its immense value as a model of modern physics, it has certain drawbacks — such as its inability to explain gravity as a fundamental force | Source: ATLAS Experiment
Oftentimes, by observing the gravitational effects of certain matter on surrounding objects, scientists can detect the existence of matter without directly observing or photographing it. Until recently, evidence supporting the existence of black holes was based on indirect observations of their massive gravitational waves. However, gravitational waves also propose the existence of a kind of “dark matter” (previously dubbed “missing mass”).
Unlike traditional matter, dark matter cannot interact with the electromagnetic force, meaning it cannot be composed of protons, neutrons, and electrons. This also means that while it does interact with gravity, it does not reflect, absorb, or emit light. Since the inferred existence of dark matter in 1933 by Fritz Zwicky, its fundamental nature and makeup have remained a mystery to physicists. The matter and forces of modern particle physics — as described by the Standard Model — cannot properly account for dark matter.
According to the Standard Model, all electrically-charged particles (all of the quarks, the electron, muon, and tau, as well as the W boson) interact with photons with the electromagnetic force. This leaves the gluon, photon, Higgs, and Z boson, as well as half of the leptons known as neutrinos.
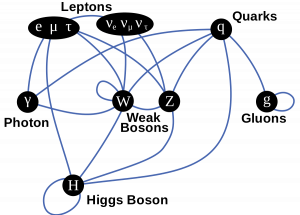
The interactions of the various fundamental particles of the Standard Model. Note how photons do not interact with any of the neutrinos, the Higgs boson, the gluon, other photons, as well as the Z boson: these particles are electrically neutral | Source: The University of Adelaide
In many aspects, neutrinos seem like the most plausible candidate for dark matter: they have non-zero masses, there exist leftover neutrinos from the Big Bang, and they only interact with the weak force — barely interacting with normal matter. In order to instigate the gravitational abnormalities associated with dark matter, the three types of neutrinos must each have a certain predicted mass. This would allow neutrinos to account for the entirety of the predicted dark matter. However, because of the difficulty in identifying and studying neutrinos directly (due to their limited interaction with most forces and matter), more indirect methods of observation can be helpful.
By analyzing the signal (result) of a \(\text{Z}\) boson (which carries the weak force) decaying in a collider, the results can sometimes be missing energy. This loss of energy in the system cannot mean energy disappeared (conservation of energy and momentum), and therefore can be an indicator of decay into a neutrino pair that simply cannot be detected by the collider sensors.
This indirect approach to neutrino detection allows for decreased uncertainty values regarding the estimated masses of the three types of neutrinos.
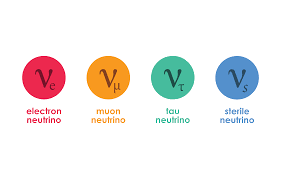
As neutrinos travel, they oscillate between each of the three known flavors (electron, muon, tau). Physicists are searching for a fourth linear superposition state known as a “sterile” neutrino with greater mass and that only reacts with gravity, potentially satisfying 100% of the mass approximations predicted for dark matter | Source: CERN
Theoretical dark matter has two basic categories: “hot” and “cold”. However, because most dark matter is “cold” (the particles move slowly relative to the speed of light), and these light neutrinos move relatively close to the speed of light, they can only account for a small fraction (approximately 1%) of the total dark matter predicted. As a result, a search for a so-called “sterile” neutrino state, which does not interact with the weak force, would account for the entirety of the predicted (cold) dark matter.
Experiments such as those at LLNL, Fermilab’s DUNE, and LSND all work on uncovering the mysteries of these elusive neutrinos, with the potential of unveiling a greater understanding of dark matter.
Be the first to comment on "A Search For Dark Matter"