Physicists from JILA, a research institute cofounded by the National Institute of Standards and Technology (NIST) and the University of Colorado Boulder, have reported developments in the use of ultracold strontium (Sr) atoms for optical lattice clocks (OLCs). By using clouds of these neutral atoms spaced a centimeter apart, along with a system of oppositely propagating lasers (dubbed an optical lattice), Zheng et al. have reported that their Sr-OLC holds atom-atom coherence times of \(26\) seconds as opposed to an atom-laser coherence time of \(96\) milliseconds while reaching a reproducible precision at the \(10^{-18}\) seconds instability level. These results suggest major implications in field applications of OLCs, reducing the dependence of instabilities on the quality of the local oscillator.
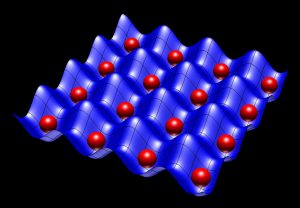
Optical lattices are formed through the perpendicular projection of interfering oscillation patterns from multiple electromagnetic wave sources. These sources, when arranged into a grid, can be used to trap atoms using principles of electron-photon emission and absorption | Source: NIST
Atomic Clocks
An atomic clock uses the interaction between excited states of various atoms and electromagnetic radiation to keep time. This electromagnetic wave, whether it be microwave or visible light, induces an energy change in the electrons, and when the energy levels transition, the interactions are recorded in relation to the electromagnetic signal. The frequency of these electronic transitions is used as a consistent, cyclical event that can, in turn, be used to keep ultraprecise time.
As atomic physicists improved their ability to manipulate these systems, the practice of laser cooling the atoms to temperatures near absolute zero has become common practice. By using the property of momentum changes in photon-atom, whether emission or absorption, interactions to homogenize the velocities (compressing the velocity distribution) of the particles, the temperature can be decreased immensely.
Laser cooling and trapping techniques have yielded dramatic increases in the accuracy of modern atomic clocks. Simultaneously, attempts at increasing the frequency and narrowing the intrinsic linewidths of the electronic transition have resulted in greater reproducible precision (usually measured in \(10^{-x}\) seconds, with an increase in significant figures implying greater precision). For example, the International Atomic Time (TAI) uses an average of global synchronized atomic clocks to a precision of \(10^{-16}\) seconds. Similarly, the Coordinated Universal Time (UTC) uses the information derived from TAI and pairs it with data of leap seconds from variations in the Earth’s rotation, yielding a more accurate global time standard.
Optical Lattice Clocks
A subset of atomic clocks, optical lattice clocks’ means of time-keeping are largely the same, with a few distinguishing characteristics in the formation of the lasers used. As the name suggests, an OLC traps a relatively large collection of neutral atoms in the interference patterns formed by a grid of oppositely propagating electromagnetic waves (known as an optical lattice). Subsequently, the atoms are exposed to a heavily tuned and stabilized frequency laser that serves the purpose of measuring the electronic transitions.
The JILA team sought to not only develop an OLC that holds accuracy to a high degree of precision but also to improve the atom-atom coherence times (essentially, a measure of how stable of a frequency the transitions are occurring) to such a magnitude, that the quality of the laser used for local oscillation decreases. The clock laser used was at \(698 \text{ nm}\) and had an intrinsic linewidth of \(\sim 1\text{ Hz}\) and linear drift rate of \(\sim 1 \text{ Hz s}^{-1}\), values marginally worse than prior industry-leading experiments yielding instabilities at \(10^{-19}\) seconds. The ultraprecise functionality of the optical lattice and strontium cloud trapping used by Zheng et al. resulted in instabilities only a single order of magnitude lower than in these past experiments. These results suggest major eases on laser precision requirements previously set, allowing for field applications of OLCs to become more realistic and cost-effective.
Applications of OLCs
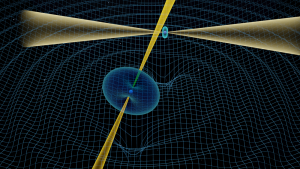
Researchers from the Commonwealth Scientific and Industrial Research Organization (CSIRO) found that a system of double pulsars interacting with one another yields the same gravitational constant behavior predicted by Einstein’s General Relativity | Source: Max Planck Institute
Ultraprecise time-keeping from OLCs developed at JILA, as well as other facilities produced by NIST or Department of Energy (DOE) laboratories, have implications in a variety of research fields. Of these, some major use cases include:
- Determining consistency of fundamental constants of interactions, such as the strength of the electromagnetic or weak nuclear force in various settings
- Demonstrating the effect of gravitational force on time through the use of relativity (through measurements of the effect of time dilation), allowing for new perspectives in relativistic geodesy (a mathematical field that researches the dimensions and topological characteristics of the Earth)
- Improving the accuracy of the Global Positioning System (GPS) by improving the onboard atomic clocks on the grid of satellites, resulting in more precise distances to be calculated by the receivers on the Earth’s surface
As photonics research becomes drastically more precise through the use of technologies such as Argonne National Laboratory’s Advanced Photon Source (APS), Fermi National Accelerator Laboratory’s Quantum Network (FQNET), and SLAC National Accelerator Laboratory’s Linac Coherent Light Source, OLCs benefit and improve proportionally. Concurrently, leaps in laser-based thermodynamic manipulation of atomic clouds also yield increasingly cost-effective and efficient results.
Be the first to comment on "Measuring Resonances: JILA Optical Lattice Clock"